Areas:
Materials/Physical/Organic Chemistry
Key Words:
Soft materials; Anisotropic colloids; Polymer networks; Directed self-assembly; DNA architectures; Colloidal crystals
We study Soft Materials, mesoscopic systems made up by polymers, colloids, emulsions, surfactants and gels etc. Examples of soft materials are plastics and rubbers, paint, shampoo, foam, and almost everything in our body including DNA, proteins, cells, tissues and muscles. A common feature shared by these diverse structural elements is that they often self-assemble into hierarchical structures driven by various non-covalent interactions. For example, biopolymers such as proteins are able to assume their native or folded state through a combination of delicate weak interactions. For synthetic systems, however, the pathway to manipulate this “interaction-structure-function” relationship remains largely undetermined.
Our research is focused on creating extraordinary soft matter systems, particularly with colloids and polymers, in which the interactions between building blocks can be modulated and even programmed. The goal is to assemble complex materials with well-defined nano- and micro-structures and therefore the desired mechanical, optical, and dynamic properties essential to important applications such as photonics, plasmonics, catalysis, energy storage, and biomedicine. Often times we draw inspirations from nature, and seek innovative synthetic, engineering, and functionalization schemes to fabricate exotic building blocks. We explore and take advantage of their collective dynamics to influence the assembling process, producing both in-equilibrium and out-of-equilibrium structures at different time and length scales.
Our projects are highly interdisciplinary, lying at the interface of Chemistry, Physics, and Materials Science, and sometimes a bit of Biology. A few of our research programs are shown below.
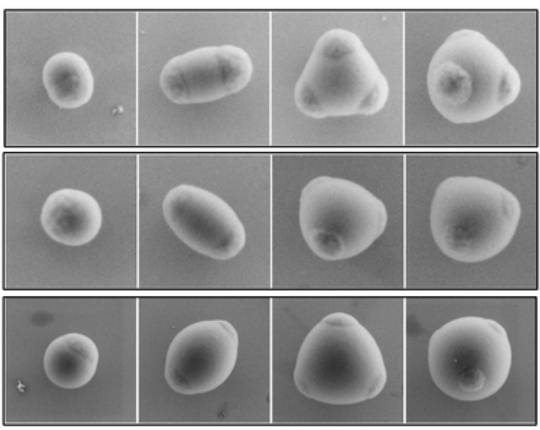
Anisotropic colloids
The ability to design and assemble 3D colloidal structures is limited using spherical particles with isotropic interactions, far from what nature can build with atoms and molecules. We explore possibilities to translate important concepts in molecular domain such as anisotropy into the word of colloids. We do so by developing strategies to synthesize colloids with various shapes, tunable sizes, and directional interactions. This leads to the creation of Janus colloids, patchy colloids, chiral colloids, reconfigurable colloids, etc., making it possible to construct a greater number of complex colloidal architectures in particular open and dynamic ones. These materials may one day find applications in photonics, catalysis, and energy storage.
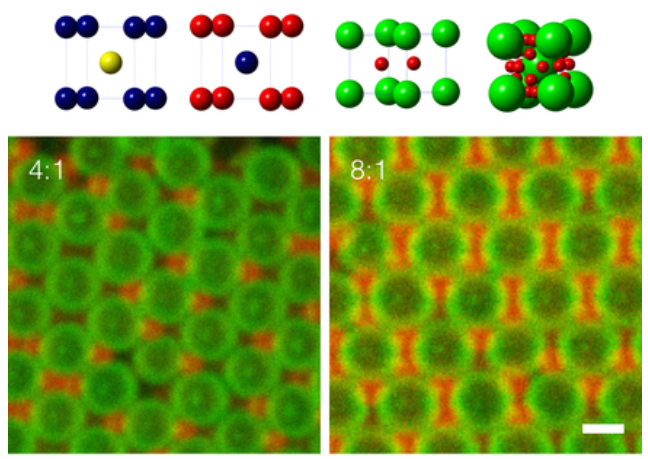
Programmable self-assembly
Programmable self-assembly is a strategy in which information necessary for assembly is encoded in the building blocks to direct the spontaneous formation of complex, yet well-defined structures. In the context of soft materials, this “information” could be the shape, size of colloidal particles, the composition or monomer sequence of polymers, or anything else that can influence the dynamics and structures of the final assembly. Once useful information is incorporated or “programmed”, the materials’ properties can be precisely predicted. We design and carry out experiments to build such systems, with information packed in their components-polymers, colloids, and nanocrystals etc.-in most cases by integrating information carrying biological molecules such as peptides and DNA. We also manipulate these systems using external fields such as electric and magnetic filed.
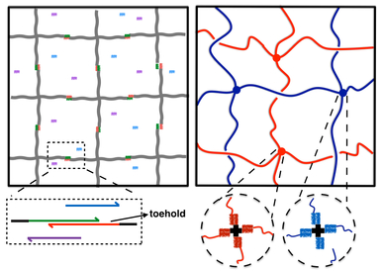
Designer Polymer networks
Within a polymer network (e.g. gels and rubbers), the nature of the crosslinks determines the connectivity of macromolecules of choice, and thus determines the ultimate materials properties. The proposed project will explore the use of information-packed oligonucleotides to construct crosslinks in synthetic polymer networks. This approach, combined with various strategies commonly employed in DNA nanotechnology, will provide a powerful means to tailor the strength, dynamics, and topology of the polymer crosslinks, assembling networks and gels unachievable before. In one example, short competing strands will be introduced to alter the bonding dynamics through strands replacement, leading to counterintuitive phase transitions. In another example, DNA nanostars or origami synthesized with well-defined shapes and bonding ability will link macromolecules of different kinds, thus producing multi-component networks for designer materials.
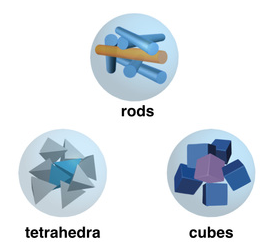